
CSIRO and the Australian National University (ANU) will display the liquid sodium receiver at the 2023 SolarPACES Conference tour of the CSIRO solar test site this October
The solar receiver on view at this year’s SolarPACES Conference site tour in October will be the first that many attendees have seen.
This solar receiver is designed for very high temperature liquid sodium as its heat transfer fluid up in a solar tower, with a solar field of heliostats in front of the tower, to reflect highly concentrated sunlight to generate higher temperatures.
In a call from Australia, Associate Professor Joe Coventry, from the Australian National University (ANU), revealed the thinking behind the choice of liquid sodium to pair with the new high efficiency power block based on the supercritical CO2 Brayton cycle.
“We’re stepping up in temperature by around about 150 degrees compared to conventional steam cycles,” said Coventry. “The supercritical carbon dioxide Brayton cycle potentially can deliver thermal-to-electric conversion efficiencies of 50%, or a bit higher; quite a step up from a steam Rankine cycle, where efficiency is around 40%. So our job was to look at technologies that would allow us to deliver heat at that sort of temperature. We had to look at what you’d use for the heat transfer fluid in the receiver.”
Liquid sodium advantages over molten salts
Operating at a higher temperature than today’s commercial CSP is a well-understood pathway to for improving efficiency, and potentially lowering the cost of electricity from CSP. But liquid sodium for heat transfer yields other advantages too.
“The design limits for this receiver are between 740 °C and 520 °C, and so that’s a 220 degrees working range,” said Coventry. “But the melting point of liquid sodium is around 100 °C. You want that as low as possible. It’s hard to find a fluid that remains liquid at very low temperatures, and yet stable at very high temperatures, like sodium.”
Molten salt has a similar working range between around 290 °C and 565 °C, but degrades at 600 degrees and solidifies and clogs the system at about 230 °C. For the high temperature sodium receiver being tested at CSIRO, there is a much bigger 300 degree buffer under its working range before freezing of the liquid become an issue. And even if it does cool that much, it is quite straightforward to gently re-melt it and get it flowing in a pipe again, unlike molten salts, according to Coventry.
For thermal storage, however, which involves a much greater volume of material, they will not use sodium, which is not particularly energy dense, and three times the cost. The prototype system at CSIRO will not incorporate storage, but there are several options for high temperature storage compatible with the sodium technology, from chloride salt mixtures to solid storage. One such ternary chloride salt is a mixture of sodium, potassium and magnesium chloride, identified by ANU, and developed further as part of the US DOE Gen3 project ‘Liquid-phase pathway to Sunshot’ project.
“That’s stable to temperatures around 750 – 800 degrees,” said Coventry. “So it has a higher high temperature stability range.” Magnesium has been on their radar at ANU. Coventry led a team at ANU that tried solid magnesia bricks in direct contact with liquid sodium in a tank with the heat transferred from the sodium to be stored in and retrieved from the magnesia bricks.
Australian research and liquid sodium
Liquid sodium is also the HTF chosen by the Australian firm Vast Energy for their new CSP/methanol solar fuel refinery under development at Port Augusta, and trialed at their 1 MW demo at Jemalong.
“It was a little cross-fertilization just sort of casually,” said Wil Gardner, who is the solar thermal engineering team leader at CSIRO. “Vast are the only people in the world that have built a sodium heat transfer fluid solar power plant since the mid-1980s. And it operated from 2014 until about 2018 as a demonstrator.”
Coventry noted that while Vast is not using it at quite as high temperature as will be demonstrated at CSIRO, ASTRI had already identified liquid metals ten years ago in looking for high temperature, high efficiency receivers.
“It’s been a parallel development of two different types of receivers, designed for different temperatures,” he said.
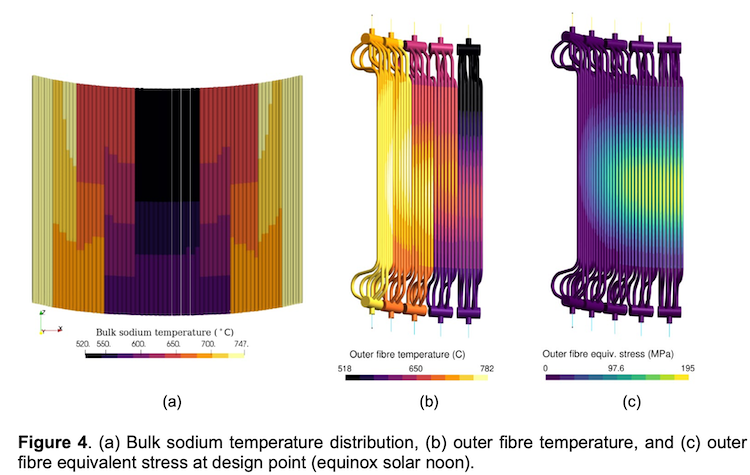
The “cold” (500 °C) sodium comes into the center panels in the receiver and then in a serpentine pattern flows up and down through five panels on each side. The tubes are bent at the top and bottom like springs to help relieve some of the stress due to thermal expansion as the receivers heat up.
“ASTRI has been interested in the sodium pathway since a scoping study in its first year, back in 2013. We shortlisted liquid metal based receivers and particle based receivers as potentially prospective to achieve high efficiency. We had an interest in a higher temperature range because of the potential to couple with high efficiency power cycles. A key differentiation between our work and the commercial development by Vast is that they’re currently targeting conventional power cycles and storage technology at temperatures lower than 600 °C. For Vast, a key benefit of sodium is that it enables the modularity of their multi-tower concept”.
The nuclear industry has used liquid sodium for heat transfer in Vast’s relatively lower temperature range for decades, making Vast’s design a commercially viable option using off-the-shelf componentry. At those temperatures it is well understood what materials to use and how to maintain sodium purity within levels that ensure corrosion is a non-issue.
Higher temperature requires new materials; more research
However, the higher temperature range being explored by ASTRI requires use of alternative materials, such as nickel-based super alloys, to maintain strength and resist creep damage. Certain metals like nickel are known to dissolve in sodium at high temperature, so understanding the most appropriate materials for this application, and how to manage issues such as corrosion will require more study.
The different temperature range impacts receiver design. At their Jemalong pilot plant, Vast used a conventional flat panel billboard receiver design for operating at temperatures in the mid five hundreds, an external receiver concept like in today’s tower CSP plants. But ANU, together with CSIRO, has developed a kind of cavity receiver for higher temperature operation.
“Cavity receivers are really good at trapping heat and have lower heat loss than externally facing cylindrical receivers,” Coventry explained.
“We’ve also worked quite hard on the optics to try and get as much light as we possibly can from the heliostats field into the receiver. For the prototype at CSIRO, we predict around about 95% of the available light that’s reflected from the heliostats will go through the aperture. We’re expecting a receiver efficiency of around 91%. So if you multiply that 95% capture by 91% receiver efficiency you get what we call a combined interception and receiver efficiency of around about 87%, which again is quite good, particularly for such a high temperature receiver. The testing that we will do at CSIRO will help see if we actually achieve these kinds of simulated numbers.”
These detailed projections can be found in the paper that ANU presented at the last conference: Design and Construction of a 700kW High-Temperature Sodium Receiver
An interesting side benefit of their cavity receiver is that there is a door that can be fully closed over the circular aperture.
“The door allows us to keep the box hot when the sun goes away so we keep the sodium heated,” he noted.
“There’s two other benefits. If there is a leak in one of the sodium tubes so there’s some smoke, at CSIRO we can contain the smoke, and we have a duct system that takes the smoke away and scrubs it out so none goes into the environment. And the other benefit is that it can shut the door against things like rain, so there’s no chance that that rain will come in and accumulate in the receiver. We’ve tested spraying water from lots of different angles at pressure and confirmed that indeed it does keep it sealed.”
What’s on the tour
The Australian Solar Thermal Research Institute (ASTRI) has been developing two alternative pathways to collect and store solar energy at high temperature to drive the new high efficiency power block based on the supercritical CO2 Brayton cycle.
The one is based on the use of liquid sodium as a heat transfer fluid, and the other based on the use of solid particles. The particle receiver, which is sharing the demo tower at the test site which CSIRO has been operating and learning from, is already up and running on site. And the sodium receiver will be on display too, but not up on the tower.
“We are 99% finished on delivering the equipment to CSIRO as built and commissioned and tested,” said Gardner.
“It won’t be up on the tower, but you can go and have a look, open up the solar receiver aperture and see inside, and a side panel will be off to allow visitors to see inside the containment vessel. So, something that you can pinch and touch and look at and stuff like that.”